Quantum entanglement is one of the great mysteries of quantum theory. When a Cesium atom gives off two photons in opposite directions, quantum theory says they become entangled and evolve as one system with net zero spin, even though each photon can still randomly spin up or down. According to quantum theory, however far apart they get, if one photon is spin up the other must be spin down. Yet if both spin randomly, how does each instantly know to be the opposite of the other?
Einstein called this “spooky action at a distance” and devised a thought experiment to deny it (Einstein, Podolsky, & Rosen, 1935). When a test was devised, based on Bell’s inequality, it showed that entanglement occurs even when the photons are too far apart to exchange a signal at the speed of light(Aspect, Grangier, & Roger, 1982). This was one of the most careful experiments ever done, as befits the ultimate test of quantum theory, and it proved quantum theory right yet again, despite there being no physical means for it to occur!
Two photons heading in opposite ways are physically apart so if each spins randomly, as quantum theory says, why can’t both be up or both be down? What connects them if not physicality? Quantum theory requires the initial zero spin to be conserved, but gives no clue as to how. Nature could conserve spin by making one photon spin up and the other down from the start, but apparently this is too much trouble. It lets both photons have either spin until one photon’s spin is registered, then instantly adjusts the other to be the opposite regardless of where the photons are in the universe. Entangled states that defy physical realism are now common in physics (Salart, Baas, Branciard, Gisin, & Zbinden, 2008).
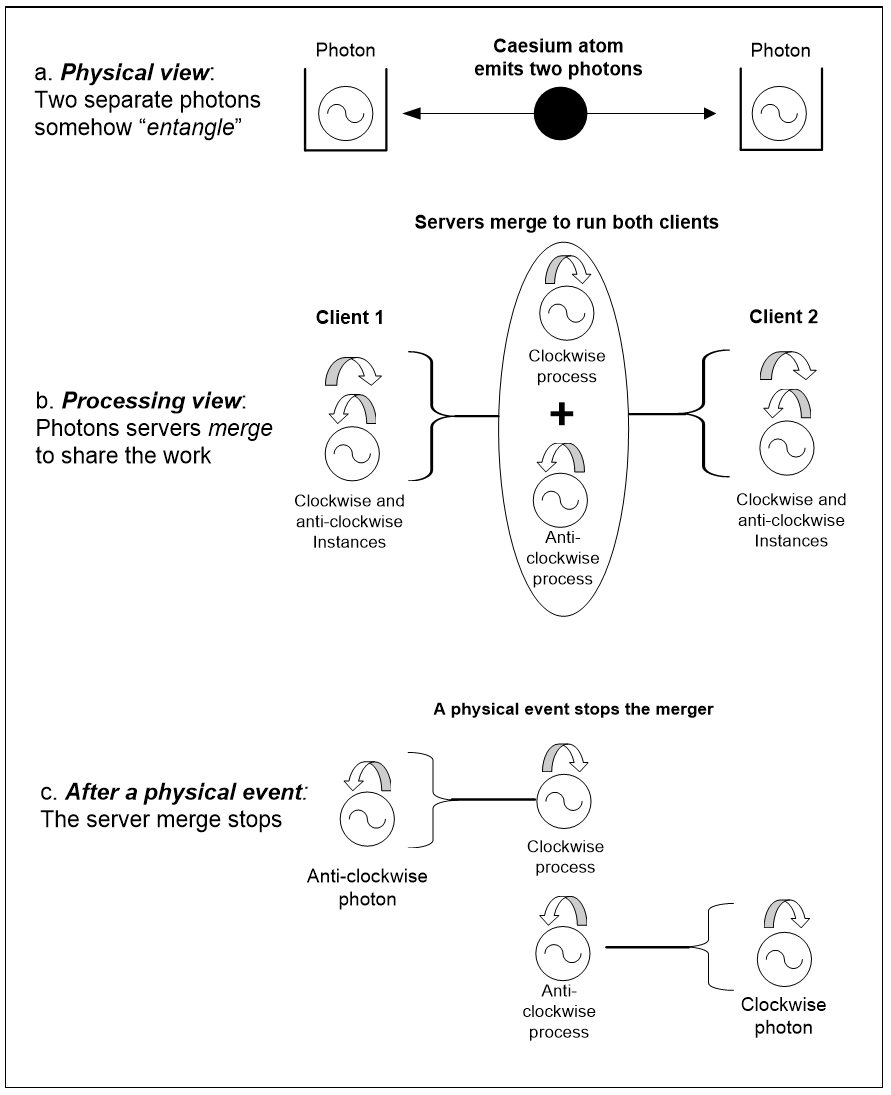
Quantum realism explains what quantum theory describes as follows. The two photons emitted by a Cesium atom begin a node reboot that reloads two photon processes at once to entangle them, and the initial net spin of both photons is zero. To us, two photons leave the Cesium atom (Figure 3.23a) but the quantum situation is more complex: the two photon servers each handle half of both photons rather than each handling one, even though they set of in opposite directions. What appears to be us as two physical photons are at the quantum level two hybrid photons handled by both photon servers (Figure 3.23b).
What entangles isn’t the physical photons but their processing, which is that of a clockwise photon and an anti-clockwise photon. The photon going left is run by two servers as is the one going right. The photons are entangled at the quantum level not the physical level. When one of the photons is observed, the instance that generates that physical event randomly restarts one photon server, leaving the opposite spin server to run the other photon (Figure 3.23c).
To recap, photons entangle when their processing merges. From that point, two servers service both “photons” jointly until another physical event starts things anew. The entangled photons look like photons and act like photons but each is two “photon halves” in server terms. Spin is conserved because the start and end processing must be the same, just as quantum mechanics requires.
Entanglement effects are non-local for the same reason quantum collapse is, that client-server relations ignore the node-to-node speed of light transfer limit. By analogy, when pixels are produced on a screen, the processing doesn’t have to “go to” a point to do that. It can change any screen point directly and likewise photon servers act directly no matter how far apart entangled photons are on the “screen” of our space.
Entanglement also underlies super-conductivity where many electrons entangle and again the server processing merges, so every electron is a processing hybrid of all the electrons. Electrons “move” with no resistance in a superconductor because each one in effect exists everywhere in the metal. In Bose-Einstein condensates any number of quantum processes can merge in this way.