The three great mysteries of science are how the universe, life and consciousness began. If a quantum event began the universe, a quantum effect began cells, and the ability to observe is a quantum property, then quantum reality could explain all three:
1. The universe began when quantum reality split into server and client (2.4.2).
2. Life began when tubulins entangled cell molecules to allow unified choices (6.3.7).
3. Consciousness always existed, so it evolved from the quantum scale (6.1.8).
There is little doubt that we and everything around us evolved from a first event billions of years ago, so the universe, life, and our consciousness are connected. The common thread is that evolution increased observation because it favors survival. If the first light became matter, matter became life, and life became us, our bodies link back to the first event. No plan was needed because, by the quantum law of all action, anything possible will eventually happen (3.6.3). Light became matter and matter became life because it is possible not because the universe is finely tuned (4.8.2). It took a long time to increase observation.
In nature, big things come from small, so our bodies grew from a cell smaller than a full stop, and bacteria we can’t see evolved into us. It follows that consciousness grew in the same way. Evolution and growth are step-wise sequences, so humans aren’t a realm apart from animals, and life isn’t a realm apart from matter. By Conway’s Free Will theorem (Conway & Koch, 2006), consciousness is all or none, so it couldn’t not exist then exist. It didn’t suddenly begin at a past moment, so even trilobites in the primeval seas observed (Figure 6.38). The ability to observe can then be traced back to the first event as follows:
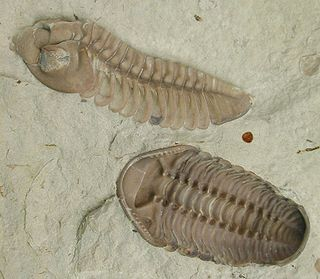
Planck time is the shortest possible time in physics. An observation at this scale would occur more times a second than there have been seconds in the life of the universe. Planck time can represent photon scale observations.
A yoctosecond (ys) is a trillion-trillionth of a second. A top quark’s lifetime is estimated at half a ys, bosons have lifetimes in ys, and quark plasma light pulses are a few ys, so this timescale may represent basic matter observations.
A zeptosecond (zs) is a billion-trillionth of a second and the shortest time measured so far. Physicists estimate a few hundred zs for the two atoms of a hydrogen molecule to photoionize (Grundmann et al., 2020), so this timescale may represent atomic observations.
An attosecond (as) is a million-trillionth of a second. Ultrafast x-ray sources with as time resolution reveal bromine molecule vibronic structures (Kobayashi et al., 2020), so this timescale may represent molecular observations.
A femtosecond (fs) is a thousand-trillionth of a second or 0.000000000000001second. It is to a second as a second is to about 32 million years. High-energy fs scale X-rays that probe complex protein molecules in light harvesting bacteria respond to light in the order of one fs (Rathbone et al., 2018) p1433, so this timescale may represent macromolecule observations.
A picosecond (ps) is a trillionth of a second or a million-millionth of a second. Estimates of coherence times for cells range from 100fs to 1 ps (Rathbone et al., 2018) p1447, so this timescale may represent simple cell observations.
A nanosecond (ns) is a billionth of a second. A billion is a big number as it takes 95 years to count to a billion. Nanosecond pulsed electric fields elicit various responses in human and other cells (Koga et al., 2019), so this timescale may represent complex cell observations.
A microsecond (μs) is a millionth of a second. Bacteria existed three billion years ago but the leap to multi-cell life was only 800 million years ago, when cell walls used ion channels that act in microseconds (Minor, 2010) p201, faster than any nerve, to let simple animals with no nerves move towards the algae they feed on (Smith et al., 2019). Microsecond pulsed electric fields of ten μs can double the growth of mushrooms exposed to them (Edwards, 2010), so this timescale may represent multicell observations.
A millisecond (ms) is a thousandth of a second. In larger animals, electro-chemical nerves replaced chemical signals. Jellyfish nerves are all over their body but oysters have a neuro-endocrine center (Liu et al., 2016). Worms and slugs have ten-thousand nerves in a chord, and crabs and insects have a hundred-thousand nerve chord. A honeybee with nearly a million nerves can fly, navigate, and communicate where pollen is. These brains are fast, as an insect startle response can be less than 5ms (Sourakov, 2011) and a praying mantis can evade a bat attack in 8ms (Triblehorn & Yager, 2005), so this timescale may represent instinctive brain observations.
A centisecond (cs) is a hundredth of a second. Frogs and reptiles have brains with tens of millions of nerves that pass data from one nerve to the next. It takes at least a cs for a signal to travel a meter of nerve, so a cerebellum-based brain can respond in hundredths of a second. Tadpole startle responses occur within 1-2cs (Yamashita et al., 2000) and our blink responses take 3-4 cs, so this timescale may represent one-center brain observations.
A decisecond (ds) is a tenth of a second. Bird and small mammal brains are about ten times larger than same-size frogs or reptiles due to midbrain and neocortex increases. Two-center brains require thalamic coherence that takes two-tenths of a second to occur, so a rats reaction time is about 2-3ds (Blokland, 1998). In 100m races, responses under a tenth of a second are considered a false start because elite sprinters take 1.2-1.6 tenths of a second to begin to move (Tønnessen et al., 2013), so this timescale may represent two-center brain observations.
The speed of thought seems to be about a second. Lower brain areas respond faster but brain-wide consciousness takes about half-a-second, so human thought will take longer. We blink in hundredths of a second and change highway lanes in tenths of a second, but it takes about a second to mentally rotate an 80° shape (Harris et al., 2000), or a 3D shape (Shepard & Metzler, 1988), or do mental arithmetic (Han et al., 2016), so this timescale may represent three-center brain observations.
Table 6.1 shows how consciousness, the ability to observe, evolved from photons to us, so the consciousness of a fly differs from ours in scale not in kind. Simpler entities observe faster so it’s hard to swat a fly that sees 250 frames a second to our 60 because, we move in slow motion compared to it. The same applies to distance, as I see a chair that it doesn’t because it sees less. Other animals have better senses but none can observe the galaxy as we do. Working back through evolution, consciousness was always there, just on a lesser scale.
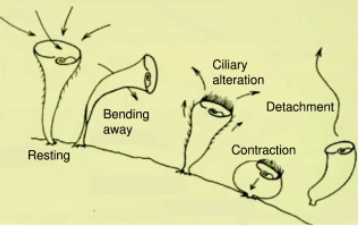
For evolution to increase consciousness it must increase survival. Eyes and wings help survival but what does consciousness do? The benefit proposed is unity. That a house divided against itself cannot stand applies as much to cells and brains as it does to societies. The brain’s binding problem was how to get trillions of nerves to act as one and a cell’s trillions of molecules have the same problem. Every composite entity has this problem – how to get its parts to work together not against each other. The benefit of unity is universal, and quantum entanglement allows it.
Unification by entanglement began with matter,as an electron is entangled photons that survive as an entity (4.3.1), atomic nuclei survive as entangled quark strings (4.6.1), and molecules survive because entanglement lets them form physically incompatible structures (3.8.1). Matter evolved by entanglement, by letting new combinations unify to be stable, and thus survive.
Unity benefits cells too, as photosynthesis works better if receptor molecules work as one, as they did when tubulin structures synchronized and entangled them. How quantum entanglement works is unclear but the benefit of unity is clear. It makes cells more than a bunch of molecules, just as it made us more than a bunch of nerve cells. For example, S. Roeselii is a trumpet-shaped single cell animal that attaches to sea rocks to feed on passing rotifers. When stimulated by an irritant, it tries various options, in order, before finally deciding to relocate elsewhere (Dexter et al., 2019) (Figure 6.39):
“They do the simple things first, but if you keep stimulating, they ‘decide’ to try something else. S. roeselii has no brain, but there seems to be some mechanism that, in effect, lets it ‘change its mind’ once it feels like the irritation has gone on too long.“
How can a cell with no brain do that? The answer proposed is that cell unity allowed cell choices that help survival. Quantum entanglement helped every step of evolution by letting complex entities make unified choices. The increase in consciousness shown in Table 6.1 is thus no accident, because the unity that consciousness provides benefits survival.
Each of us is a walking, talking, thinking complex of 30 trillion cells that grew from one cell by a path that evolution discovered. We see ourselves as uniquely conscious, but evolution doesn’t do unique. We are only conscious because countless life forms before us found ways to become more so. The vast tree that bore us stretches as far as we can see and more, but how does it exist?
Table 6.1 The Evolution of Consciousness |
|||
Observer |
Time Scale |
Examples |
|
Light |
Planck time |
̴10−44 seconds |
Photon |
Basic matter |
Yoctosecond |
10−24 seconds |
Electrons, quarks, neutrinos |
Atoms |
Zeptosecond |
10−21 seconds |
Periodic table atoms. |
Molecules |
Attosecond |
10−18 seconds |
Oxygen, carbon dioxide … |
Macromolecules |
Femtosecond |
10−15 seconds |
DNA, RNA, mtDNA |
Simple cells |
Picosecond |
10−12 seconds |
Bacteria and organelles |
Complex single cells |
Nanosecond |
10−9 seconds |
Paramecium, amoeba |
Multicell life |
Microsecond |
10−6 seconds |
Placozoa, algae, fungi |
Instinctive brains |
Millisecond |
10−3 seconds |
Fish, insects, crabs |
One-center brains |
Centisecond |
10−2 seconds |
Reptiles, amphibia |
Two-center brains |
Decisecond |
10−1 seconds |
Mammals, birds |
Three-center brains |
Seconds |
Seconds |
Humans |