Uncategorized
Chapter 2 References
Ambjorn, J., Jurkiewicz, J., & Loll, R. (2008). The Self-Organizing Quantum Universe. Scientific American, 299 July(1), 24–31.
Barbour, J. (1999). The End of Time: The next revolution in physics. Oxford University Press.
Barrow, J. D. (2007). New theories of everything. Oxford University Press.
Berners-Lee, T. (2000). Weaving The Web: The original design and ultimate destiny of the world wide web. Harper-Collins.
Campbell, T. W. (2003). My Big TOE (Vol. 3). Lightning Strike Books.
Cartwright, J. (2020). We may have spotted a parallel universe going backwards in time. New Scientist, 8 April.
Chadwick, H. (2008). St. Augustine, Confessions. Oxford University Press.
Cho, A. (2000). Physicists Unveil Schrodinger’s SQUID. Science, 287(31 March).
Chopra, D. (1989). Quantum Healing. Penguin.
Cliff, H. (2015). Have we reached the end of physics? Dec.
Cole, K. C. (2001). The hole in the universe. Harcourt Inc.
Collins, G. P. (2006). Computing with quantum knots. Scientific American, April, 56–63.
Crane, L. (2020). Neutrinos may explain why we don’t live in an antimatter universe. 15 April.
Davies, P. (2004). Emergent Biological Principles and the Computational Properties of the Universe. Complexity, 10(2), 11–15.
Davies, P. (2006). The Goldilocks Enigma. Penguin Books.
Davies, P., & Brown, J. R. (1999). The Ghost in the Atom. Cambridge University Press.
Dawkins, R. (1989). The Selfish Gene (Vol. 2nd). Oxford University Press.
D’Espagnat, B. (1995). Veiled Reality: An analysis of present-day quantum mechanical concepts. Addison-Wesley Pub. Co.
Einstein, A. (1920). Ether and the Theory of Relativity. http://www-history.mcs.st-and.ac.uk/Extras/Einstein_ether.html
Fredkin, E. (2005). A Computing Architecture for Physics. Computing Frontiers 2005, 273–279.
Greene, B. (2004). The Fabric of the Cosmos. Vintage Books.
Guth, A. (1998). The Inflationary Universe: The Quest for a New Theory of Cosmic Origins. Perseus Books.
Hartle, J. B. (2005). The Physics of “Now.” Am.J.Phys., 73, 101–109, avail at http://arxiv.org/abs/gr-qc/0403001.
Hawking, S., & Penrose, S. (1996). The nature of space and time. Princeton University Press.
Hawking, S. W., & Hartle, J. B. (1983). The basis for quantum cosmology and Euclidean quantum gravity. Phys. Rev., D28(2960).
Hertog, T. (2024). On the Origin of Time. Penguin.
Heylighen, Francis, & Chielens, K. (2009). Evolution of Culture, Memetics. In Meyers, B (Ed.), Encyclopedia of Complexity and Systems Science. Springer. http://en.wikipedia.org/wiki/Memes
Hossenfelder, S. (2018). The Present Phase of Stagnation in the Foundations of Physics Is Not Normal. Nautilus, November. https://nautil.us/the-present-phase-of-stagnation-in-the-foundations-of-physics-is-not-normal-237258/
Hoyle, F. (1950). The Nature of the Universe. Harper & Brothers – New York.
Jenner, N. (2014). Five Practical Uses for “Spooky” Quantum Mechanics. Smithsonian Magazine, December 1.
Kauffman, S., & Smolin, L. (1997). A possible solution to the problem of time in quantum cosmology. arXiv Preprint, http://xxx.lanl.gov/abs/gr-qc/9703026, 1–15.
Kragh, H. (1990). Dirac: A Scientific Biography. New York : Cambridge University Press.
Kuhn, T. (1970). The Structure of Scientific Revolutions: Vol. Second Edition, Enlarged. The University of Chicago Press.
Mazur, J. (2008). Zeno’s Paradox. Penguin Books.
McCabe, G. (2005). Universe creation on a computer. Stud.Hist.Philos.Mod.Phys.36:591-625.
Penrose, R. (2010). Cycles of Time. Vintage Books.
Petersen, A. (1963). The Philosophy of Niels Bohr. Bulletin of the Atomic Scientists, 19, 8–14.
Randall, L. (2005). Warped Passages: Unraveling the mysteries of the universe’s higher dimensions. Harper Perennial.
Randall, L., & Sundrum, R. (1999). An Alternative to Compactification. Phys.Rev.Lett., 83, 4690–4693.
Richard Feynman. (1985). QED, The Strange Theory of Light and Matter. Princeton University Press.
Sandburg, A., & Bostrom, N. (2008). Whole Brain Emulation: A Roadmap. Future of Humanity Institute, Oxford University, Technical Report #2008‐3.
Seibt, J. (2024). Process Philosophy. In The Stanford Encyclopedia of Philosophy (Spring). Stanford University Press.
Shannon, C. E., & Weaver, W. (1949). The Mathematical Theory of Communication. University of Illinois Press.
Smolin, L. (2006). The Trouble with Physics. Houghton Mifflin Company.
Sutter, P. M. (2022). Why We Need to Study Nothing. Nautilus, August 2.
Walker, E. H. (2000). The Physics of Consciousness. Perseus Publishing.
Watson, A. (2004). The Quantum Quark. Cambridge University Press.
Wheeler, J. A. (1989). Information, physics, quantum: The search for links. Proceedings III International Symposium on Foundations of Quantum Mechanics, 354–358.
Whitworth, B. (2008). Some implications of Comparing Human and Computer Processing.
Whitworth, B., & deMoor, A. (2003). Legitimate by design: Towards trusted virtual community environments. Behaviour & Information Technology, 22(1), 31–51.
Wilczek, F. (2008). The Lightness of Being: Mass, Ether and the Unification of forces. Basic Books.
Woit, P. (2007). Not even wrong. Vintage.
Wootters, W., & Zurek, W. (1982). A Single Quantum Cannot be Cloned. Nature, 299, 802–803.
Chapter 2 Questions
The following questions are addressed in this chapter. They are better discussed in a group to allow a variety of opinions to emerge. The relevant section link is given after each question:
1. Can information be defined in purely physical terms? Do so, or explain why it isn’t possible. (QR2.1.2)
2. Does a hologram of past physical events replay reality? What is missing? (QR2.1.3)
3. Can one copy a physical state? What about a physical event? What about a quantum state? (QR2.1.4)
4. How does quantum processing differ from physical processing? Why is it so powerful? (QR2.1.5)
5. If the physical world is digital what is its resolution and refresh rate? (QR2.2.1)
6. State Zeno’s paradoxes. How does physics resolve them? What does resolve them? (QR2.2.1)
7. Is space something or nothing? If nothing, what transmits light? If something, what is it? (QR2.2.2)
8. Would a network generating our universe be centralized or distributed? Explain why. (QR2.2.4)
9. Why do circular dimensions explain our space better than linear dimensions? (QR2.2.5)
10. How can space expand everywhere at once, as physics says? (QR2.2.6)
11. State one disadvantage of a polar space. Why doesn’t it matter for our space? (QR2.2.7)
12. What does a dimension outside our space imply that one curled up within it doesn’t? (QR2.2.8)
13. If reality has a fourth dimension, why can’t we enter it? (QR2.2.8)
14. If light is a transverse wave, like a wave on a lake, on what surface is it vibrating? (QR2.2.9)
15. Traveling at near light speed slows down time, so would you live longer? (QR2.3.1)
16. Is there any evidence for time travel in physics? Why is it unlikely? (QR2.3.2)
17. Why can’t quantum entities go back and forth in time? (QR2.3.3)
18. Is there an alternative to the block theory of time? What is it? QR2.3.4)
19. Why is cosmic background radiation from the early universe still all around us? (QR2.4.1)
20. What caused the initial inflation of the universe and what stopped it? (QR2.4.2)
21. What happens if a data transfer in a simulation fails? How do our systems avoid this? (QR2.4.3)
22. How could a quantum network avoid transfer failures? (QR2.4.4)
23. Is the vacuum of space empty or full, and if full, what is it full of? (QR2.4.5)
24. Why is theoretical physics no longer advancing? (QR2.5.1)
25. What can science do when a theory no longer generates new knowledge? (QR2.5.2)
26. Do the equations of quantum theory describe what is imaginary or real? Justify. (QR2.5.3)
27. Why do physicists call what quantum theory describes imaginary when it predicts physical events? (QR2.5.3)
28. Is quantum realism a God theory? Why or why not? (QR2.5.3)
29. If quantum waves are processing waves, what is a physical event? (QR2.5.4)
QR2.5 Re-engineering Physics
Last century, the equations of physics were one-liners, like E=mc2, but the equations of string theory today fill books. The low-hanging fruits of nature’s tree of knowledge have been plucked it seems. The ladder of machine learning was expected to help, but thirty years of data crunching didn’t alter the situation. Meanwhile, the theory front produced one wrong prediction after another, for as a physicist notes:
“Ten thousand wrong predictions sounds dramatic but its actually an underestimate… all the extra dimensions … all the pretty symmetry groups, all the new particles … They were all wrong. Even if the LHC finds something new in the data that is yet to come, we already know that the theorist’s guesses did not work out. Not. A. Single. One. How much more evidence do they need that their methods are not working?” (Hossenfelder, 2018).
Fifty years without progress in physics is unprecedented, so it’s time for a change. The change proposed now is to stop spinning what Hossenfelder calls theory-tales, and re-engineer physics from the data ground up.
QR2.5.1 The End of Physics?
QR2.5.2. Grounded Physics
QR2.5.3. Reality Is Quantum
QR2.5.4. A Quantum Model
QR2.4.5 Empty Space is Full
If only matter exists, the space between objects should be nothing at all. Nothing should do nothing, but we know that the vacuum transmits light, gravity, magnetism, and charge, and that isn’t nothing. It is said that from nothing, nothing comes, but from our space comes the distance between objects, for if nothing separated objects in empty space they would be touching! A space that transmits waves and separates objects can’t be nothing.
The Casimir effect illustrates this, as uncharged plates close together in a vacuum register a force pushing them together (Cole, 2001). Quantum theory predicts this pressure but a truly empty space shouldn’t do this. Martin Reece, Astronomer Royal and Emeritus Professor of Cosmology and Astrophysics at Cambridge University, concludes that space isn’t just the absence of matter.
“We know that the universe is very empty. The average density of space is about one atom in every ten cubic metres – far more rarefied than any vacuum we can achieve on Earth. But even if you take all the matter away, space has a kind of elasticity which allows gravitational waves – ripples in space itself – to propagate through it. Moreover, we’ve learned that there is an exotic kind of energy in empty space itself.” (Martin Reece).
Space looks like nothing but is actually something, so how can that be? The current reply is field theory, that light travels by vibrating a field within space in a direction outside space, as Maxwell’s equations describe. In effect, space hosts an invisible field that vibrates in an invisible direction to cause light. This is accepted because the equations work, but how can a physical field vibrate outside space? Field theory also lets virtual particles from this field push the Casimir plates apart, but what creates those particles? Field theory essentially allows empty space to have physical effects, as it does, but how can nothing host a field that generates particles?
The alternative now proposed is that the quantum network shows something or nothing as a screen shows an image or blankness. Empty space is then a screen null value, light is positive-negative values spreading, and matter is a constant positive or negative value. The following chapters give more detail, but basically this gives a single cause for space, light, and matter, namely the quantum network. It also lets space create distance, so the earth doesn’t touch the moon because the null points of space separate them.
Why then does empty space have energy? If empty space is null processing, why isn’t the result all zeros? The answer is that null processing isn’t constantly null. A positive-negative null process is only zero when the cycle ends, so at any moment, some points in a region will be zero but others won’t. Points will average zero over time but for an asynchronous network, as proposed here, they aren’t all zero at once. Empty space isn’t always empty, just as a blank TV screen can show static. Quantum theory doesn’t allow space be constantly null, so:
“… space, which has so much energy, is full rather than empty.” (Bohm, 1980), p242.
That space is something not nothing, and that it transmits light waves, implies a non-physical ether. Einstein also concluded long ago that some sort of ether had to exist for relativity to work:
“…there is a weighty argument to be adduced in favour of the ether hypothesis.” (Einstein, 1920).
Strangely enough, modern field theory now supports the idea of a non-physical ether:
“The ether, the mythical substance that nineteenth-century scientists believed filled the void, is a reality, according to quantum field theory” (Watson, 2004), p370.
For Newton, space was a static tablecloth that presented objects like cutlery, but field theory sees it as an ocean that spits quantum foam and particles that jump like fish. But if empty space is full, what is it full of? Physical realism can’t say, but quantum realism lets it be full of quantum processing.
When one looks out a window, one sees the view not the glass transmitting it. We know a glass is there if it is imperfect, if there is a frame around it, or if it can be touched. Now suppose that our window on reality has no imperfections so it can’t be seen, is all around us so it has no frame, and accommodates matter so it can’t be touched. It is like a perfect glass that reveals reality but doesn’t show itself. It is perfectly clear, with no flaws or imperfections, so it can’t be seen. It is boundless, without sides or edges, so it can’t be seen around. And it accommodates matter, so it can’t be touched. We walk, talk, and act within what we can’t register, so we call it empty space, but actually what we call empty is full.
QR2.4.4 The Pass-it-on Protocol
The transfer protocol now proposed is staggeringly simple. It is that quantum waves are passed on as soon as they are received, so they spread like ripples in a pool. A pebble that falls onto a pool pushes the water down until it rebounds, and that up-down displacement spreads as ripples on its surface. Likewise, a photon starting on a quantum network surface is also an up-down displacement passed on, giving the quantum ripples we call light.
If quantum waves are processing waves, each point involved has to do two things: run the process and pass it on. One might expect it to run the process first then pass it on but to avoid transfer losses, it is better to pass the processing on as soon as it is received. The pass-it-on protocol is then that any processing received is passed on immediately, to ensure that it isn’t lost.
If a transfer sent to a point waited for it to finish what it was doing, the speed of light would vary for the same route, but it doesn’t. This implies that each transfer is received as an interrupt, which in computing is a signal that has priority over anything else a processor is doing. For example, in Windows, pressing Ctrl-Alt-Del keys together interrupts the CPU to run the Task Manager.
The pass-it-on protocol essentially prioritizes transfers over anything else, so the speed of light is constant because light waves are always immediately passed on. Light spreading throughout the universe also helps synchronize the network, despite it being decentralized. The effect isn’t perfect, but light interrupting points everywhere at a constant rate increases synchrony.
A weakness of this protocol is that transfers in a circle would give an endless interrupt loop that reduces processing, like the deadlock loop earlier. Fortunately, space expanding mitigates this, as new points of space have nothing to do for their first cycle. This reduces the loop build-up, but that a halo of light circles the galaxy center could explain dark matter (4.7.6).
In this protocol, nothing ever waits, so it is efficient, no transfers are lost, so it is reliable, and quantum waves are generated, so it is effective. It works well but if it did fail, even for a moment, not only our universe, but also its evolution, would be gone forever. The only recovery then would be to restart another universe from scratch.
QR2.4.3 Transfer Errors
Our networks need protocols to avoid transfer errors, so how does the quantum network handle this? Transfer errors are when network data is sent but not received, like when a catcher drops a ball from a pitcher. For example, if one point sends data to another when it is busy, the transfer is lost, just as a ball thrown when the catcher isn’t ready is dropped. Equally, if two points transfer to the same point at the same time, one is lost, just as if two pitchers throw two balls to a catcher at the same time, catching one means the other is lost.
But does it matter if a transfer is lost, as after all, it’s just information? Unfortunately, the money in your bank account is also just information, so a failed bank transfer could lose it all! If two people call you at once, it may not matter that one gets a busy signal, but on a network, losing a transfer loses what it represents. In a virtual world like Sim City, objects are represented by information, so a failed transfer could make the sword in your hand suddenly disappear, so networks can’t afford to lose transfers.
Our universe has, as far as we know, conserved energy for billions of years, so if the movement of every photon in our universe is a transfer, none have been lost, or we would notice it. If our world is virtual, the network generating it must avoid transfer errors, but how? Our networks avoid transfer errors by protocols like:
1. Locking. Makes a receiver exclusively available before sending the transfer.
2. Synchrony. Synchronizes all transfers to a common clock.
3. Buffers. Stores transfer overloads in a buffer memory.
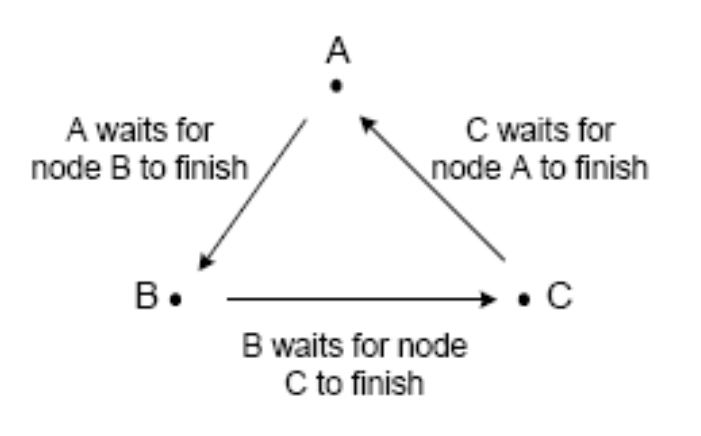
Could a quantum network use any of these methods?
Locking. Locking makes a receiver dedicate itself to a sender before the transfer is sent. For example, if I edit this document, my laptop locks it exclusively, so any other edit attempt fails, with a message that it is in use. Otherwise, if the same document is edited twice, the last save will overwrite the changes of the first, which are lost. Locking avoids this by making every transfer two steps not one, but also allows transfer deadlock (Figure 2.13), where point A is waiting to confirm a lock from B, that is waiting for a lock from C, that is waiting for a lock from A, so they all wait forever. If the quantum network used locking, we would occasionally find dead areas of space unavailable for use, but we never have, so it can’t use locking to avoid transfer losses.
Synchrony. On a computer motherboard, when a fast central processing unit (CPU) sends data to a slower memory register, it must wait before sending another transfer. If it sends data again too soon, the transfer fails because the register is still busy with the first transfer. On the other hand, if it waits too long, this wastes valuable CPU cycles. It can’t check if the memory is free before sending because that is a new command that also needs checking! Using the double-send of locking would slow the motherboard down, so it uses a common clock to synchronize events. The CPU sends data to memory when the clock ticks, then sends more data when it ticks again. This avoids transfer losses if the clock is set to the speed of the slowest component, plus some slack. One can increase a motherboard’s clock rate, to make it run faster, but too much over-clocking will crash it. Synchrony requires a common time but according to relativity, our universe doesn’t have that. If the quantum network had a central clock, it would cycle at the rate of its slowest part, say a black hole, which is massively inefficient, so it can’t use synchrony to avoid transfer losses either.
Buffers. The Internet uses memory buffers to avoid transfer errors so it can be decentralized. Protocols like Ethernet (Note 1) distribute control, to let each point run at its own rate, and buffers handle the overloads. If a point is busy when a transfer arrives, a buffer stores it to be processed later. Buffers let fast devices work with slow ones. If a laptop sends a document to a printer, it goes to a buffer that feeds the printer in slow time, so you can carry on working while the document prints. But buffers require planning, as too big buffers waste memory and too small buffers can overload. Internet buffers are matched to load, so backbone servers like New York have big buffers, but backwaters like New Zealand have small ones. In our universe, a star is like a big city while empty space is a backwater. If the quantum network used buffers, where stars occur would have to be predictable, which according to quantum theory, wasn’t so. Equally, same buffers in the vastness of space would be a massive waste of memory, so it can’t use buffers to avoid transfer losses either.
Our networks avoid transfer losses by locking, synchrony, or buffers, but quantum events proceed one step at a time so the quantum network can’t use locking, our universe has no common time so it can’t use synchrony, and the quantum network has no memory storage so it can’t use buffers. The quantum network can’t wait for locks or clocks, and has no buffer memory, so how can it avoid transfer errors?
Note 1 . Or CSMA/CD – Carrier Sense Multiple Access/ Collision Detect. In this decentralized protocol, multiple clients access the network carrier if they sense no activity but withdraw gracefully if they detect a collision.
QR2.4.2 The Little Rip
Based on how fast our universe is expanding, scientists were able to rewind back to when it began. Big bang theory is that about 14 billion years ago our entire universe existed at a point that exploded out to become what we see today, but for this to be true, several miracles had to happen.
The first was that everything in our universe had to come from nothing. It is a miracle because from nothing, nothing comes, so how can a universe come from it? This is the something from nothing miracle.
The second miracle was that our entire universe initially existed at a point, called a singularity. It is a miracle because by the laws of physics, matter at that density should immediately collapse into a black hole, from which even light can’t emerge, so the universe would be stillborn. This is the singularity miracle.
To avoid this, Guth proposed inflation theory (Guth, 1998), that an immense anti-gravity field expanded the singularity faster than light for 10-32 seconds, so it didn’t become a black hole. He solved one miracle by proposing another, because according to relativity, nothing can move faster than light. This is the faster than light miracle.
This huge field then vanished as suddenly as it appeared, for no reason, to play no further part in our universe. It is a miracle because no other fields of physics have ever vanished. This is the vanishing field miracle.
Instead of an all-powerful being creating the universe, physics now says that it came from nothing, to exist at a single point, that a massive field expanded faster than light, until that field vanished forever, leaving the universe to slowly evolve into galaxies, stars, and us. But how is trading one miracle for four an improvement, despite its scientific veneer? Is there any theory that avoids these miracles?
Quantum realism begins by stating that quantum waves exist, based on the evidence. A universe that began must begin from something, so the network that hosts those waves could have existed before our universe began, to start it. But how can one reality give rise to what isn’t itself? One way, for a network, is to create a virtual reality based on server-client relations.
A server-client relation is when one point of a network tells another what to do. For example, if I print this page, my laptop tells the printer what to print, so it is a server and the printer is a client. If a client job fails, the server can restart it so my laptop can resend the page if the print fails. Likewise, if a quantum wave is client events spreading on a network, it can restart again as quantum theory says. A server directing a quantum wave can restart it if it fails just as I can restart a print that fails. That the physical world began as server-client waves then avoids the something from nothing miracle.
Our universe as a server-client virtual reality should begin as our software environments do. When a Windows computer is turned on, it first loads a tiny CMOS program, that then loads a kernel program, that loads a bigger BIOS, that loads the full Windows environment, so the software starts in a step-wise manner, not all-at-once. If our universe did the same, the first event only needs to create one photon to start it off. That our universe began as one photon then avoids the singularity miracle, because one photon won’t collapse into a black hole.
To create a photon, which is one quantum wave, only requires one network point to give its activity to its neighbors, to became a server. Doing this would leave a Planck size hole in the network, so space also began when our universe did. This white-hot speck of light could then trigger other points to do the same, just as a little rip in a taught fabric quickly becomes big. Inflation was then the quantum fabric dividing itself into servers and clients, to give an initial plasma that was:
“… essentially inhabited by massless entities, perhaps largely photons.” (Penrose, 2010), p176.
In our networks, server events are faster than client events because directing an event is faster than doing it. Hence, if the initial creation of photons was a server chain-reaction, it would occur faster than the speed of light, which reflects the client rate of network transfer. That inflation was a server chain-reaction then avoids the faster-than-light miracle, but why didn’t it continue forever?
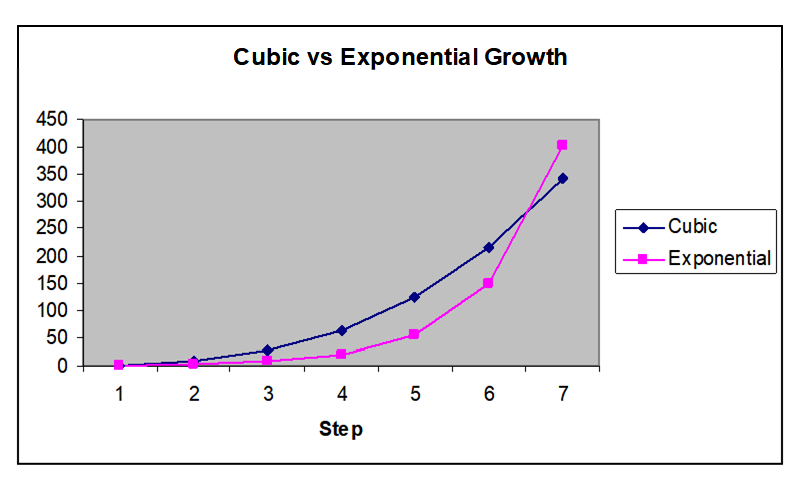
In the above, each step of the inflation chain-reaction produced both light and space. Adding space extended the wavelength of light to dilute its energy, so light that was white-hot at the dawn of time is now cold. The generation of light was exponential, as light begat light, but the hypersphere surface of space grew as a cubic function, which overpowers exponential growth if the resolution is quick (Figure 2.12), as physics says it was (Note 1). That space expanding stopped inflation by cooling the light causing it then avoids the vanishing field miracle.
It follows that the big bang wasn’t big, at first anyway, nor was it a bang, as initially there was no space to explode into. It was a little rip in the primal reality that made both light and space. The quantum network separated itself into servers and clients to create the quantum waves that cause physical events, so our universe was borne not manufactured. Space expanding then healed the rip, but not before all the photons in our universe had been created. In this view, our universe began as nothing but white-hot light, and matter came later, as Chapter 4 explains. After the initial act of creation, space continued to expand, cooling the universe down, so space expanding isn’t just an oddity of physics. We only exist because it cooled the universe enough to accommodate us.
In conclusion, our universe came from something not nothing, it was a little rip not a big bang, followed by an outward push not a pull, that was stopped by the expansion of space not a field disappearing. No miracles are needed, given only that quantum waves exist.
Note 1. In inflation theory, an immensely strong anti-gravity field pulled the entire physical universe from the size of a proton to the size of a baseball faster than the speed of light, then 10-32 of a second later that field conveniently disappeared forever.
QR2.4.1 Space is a Surface
In 1929, the astronomer Hubble observed that all the stars and galaxies were speeding away from us, so our universe is expanding everywhere. But if so, why is light from just after the big bang still all around us today, as cosmic microwave background? If the early light exploded outwards, shouldn’t it be at the edge of the universe not here? Current physics doesn’t explain this, but space as an expanding surface does (2.2.5).
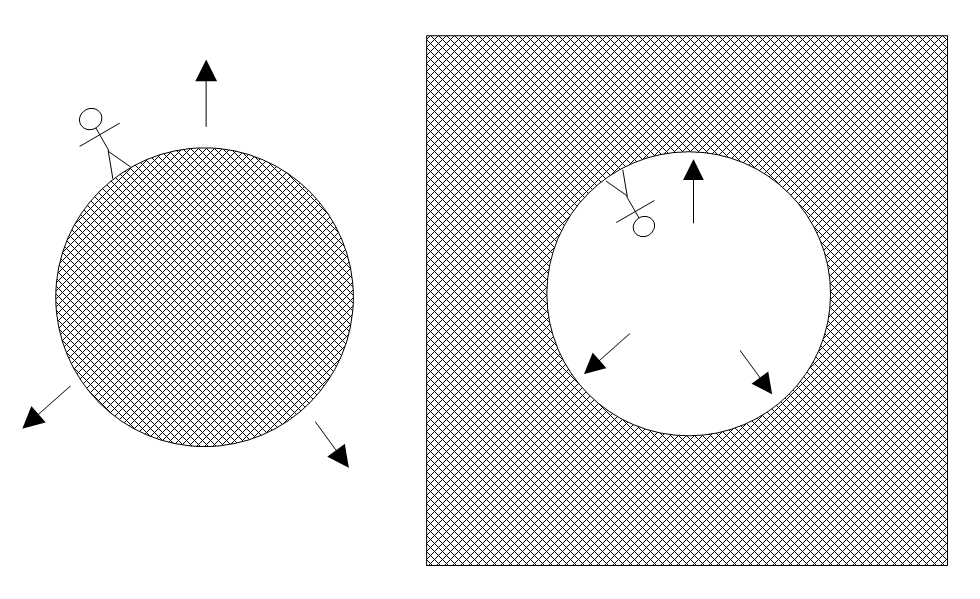
A hypersphere surface has three dimensions like our space and no edges like a balloon surface, so it lets our space expand everywhere at once, not just at an edge. Yet while a balloon expanding thins out, our space hasn’t changed in billions of years. This suggests that we are on the inside surface of expanding bubble, not the outside of a stand-alone balloon (Figure 2.11). The surface of a bubble in a liquid doesn’t thin out as it expands because its surface comes from the liquid, not itself. It still has no center or edge, so any waves on it will wrap around to end up everywhere. Hence, light from long ago is still all around us, as cosmic microwave radiation. It also answers questions like:
- What is space expanding into? It is expanding into the bulk around it.
- Does space end? No, a bubble surface has no end.
- Where is space expanding? Everywhere, just as a bubble surface expands everywhere at once.
- How does new space arise? As the bubble expands, new space comes from the bulk around it.
- Are we expanding too? No, objects on a bubble surface don’t expand as the bubble does.
Space as an expanding bubble surface is homogenous but not continuous, expanding but not exploding, never-ending but not infinite, and curved not flat. Instead of hosting particles in space, it only hosts waves on space, so our universe is built from waves not particles.